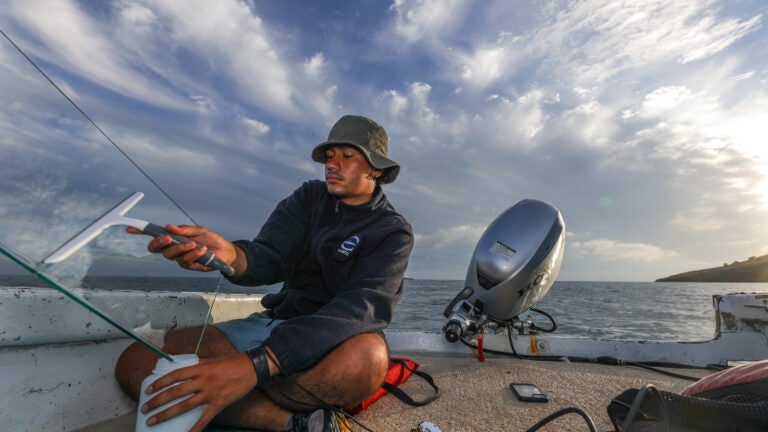
Sea surface micro-layer sampling technique, using a quartz plate (Photo by Yannick Peterhans/WIES)
Sun loving bacteria in the ocean-air interphase
I honestly never thought I would be back to fisherman’s hours in grad school. But I am always grateful to the ocean for taking care of me all these years.
As the first rays of light hit our window, David (my undergrad helper for the summer) and I are already awake, preparing to head out to the lab. As we rinse all the equipment that we need to take down the hill to the Loper (our boat), I can hear the voice of my abuelo saying, “Mijo usted tiene que aprender a hacer de todo en esta vida, incluso a lustrar zapatos por que uno nunca sabe cuando le va a server,” which roughly translates to “No skill is ever useless, no matter how simple it might seem.”
My grandpa’s words start to make even more sense as I drive the boat offshore to the sampling spot designated for this cycle, drop the anchor, measure all the environmental parameters, and start cleaning windows in the middle of the ocean to sample for the sea surface microlayer—using not only the knowledge from my undergrad years, but also what I learned years ago on the Colombian coasts and streets where I grew up.
Hola! My name is Danny Alejandro Osorio, and I’m a second-year Ph.D. student in USC’s Marine and Environmental Biology department working with Dr. Laura Gomez Consarnau. I am also the North American coordinator for a United Nations YOUNGO (youth climate organization) working group called Oceans Voice. I work closely with South American non-governmental organizations (NGOs), like pacto x el clima, where I advocate for sustainable fisheries and marine life protection through policies that don’t harm small coastal communities like my hometown. My research at our home lab revolves around a pathogenetic bacteria species known as vibrio. More broadly, our lab studies a specific pigment, called Proteorhodopsin, that is found on most marine bacteria.

Pigments are fundamental to photosystems, as they allow organisms to convert sunlight into biologically useful energy (a process called phototrophy). A familiar example is chlorophyll, the pigment found in terrestrial plants that causes them to be green in color. Chlorophyll enables photosynthesis. This same pigment is responsible for photosynthesis in the open ocean. There, the process mostly occurs in single-cell organisms, like bacteria and phytoplankton. Most of the oxygen that we breathe comes from this process.
Proteorhodopsin (PR) photosystems are light-driven proton pumps that enable synthesis of ATP in heterotrophic bacteria, potentially making them less dependent on organic matter sources in the ocean. (ATP is the molecule that provides energy to cells, and heterotrophic organisms eat other plants or animals for energy.) This type of phototrophy is widespread among surface marine bacteria (those that live at depths of 1-3 meters), with over 80% of all bacterioplankton genomes containing the necessary genes for this function. These data challenge the notion that chlorophyll-based photosynthesis is the primary light-energy harvesting mechanism in marine microbial organisms.
PR variants are not distributed randomly in the water column but dispersed along its depth. This is largely due to how light travels through water. Recent studies in our lab show that PR concentrations are highest at near-surface depths, suggesting that bacteria with this light-capturing mechanism are less affected by light saturation and can take full advantage of the highest light intensities available. A missing piece to our understanding of the distribution of PRs in aquatic environments is understanding PR presence in the surface microlayer (SML). The sea surface microlayer is the boundary between the atmosphere and the ocean. It covers 70% of Earth’s surface and is the interface where the exchange of heat, momentum, and mass takes place between the ocean and the atmosphere. However, even with all its importance, this area is poorly understood and rarely represented in environmental models. This is because its thickness is only around 1mm and is very hard to sample. To sample the SML, David and I have to place a quartz plate in the water and scrape what attaches to it into collection vessels, similar to the process of using a squeegee to clean windows. It takes us about 3 hours to collect approximately two liters of water through this process.

The main goal of my summer research at Catalina Island is to study, for the first time, PR distribution in the ocean’s SML. Why is this important? These data will allow us to determine PR distribution throughout the water column With this information, we can potentially create more precise models of how other organisms further down the water column—such as our beautiful kelp forest—are processing light. In addition, microorganisms are the drivers of most of the biochemical processes of the ocean. Knowing how they are distributed, and which strains of bacteria are present, is a great tool to avoid disease outbreaks, understand how energy travels through the water, and assess the overall state of our oceans.
It’s still too early to report any findings, as we are still collecting samples, but I am excited to process all my samples and subsamples to give us a complete picture of the difference between the SML and sub-surface water off the coast of this side of Catalina Island. I can’t express in words how grateful I am for the opportunity Wrigley has given me, and I hope it can be helpful for future environmental models.