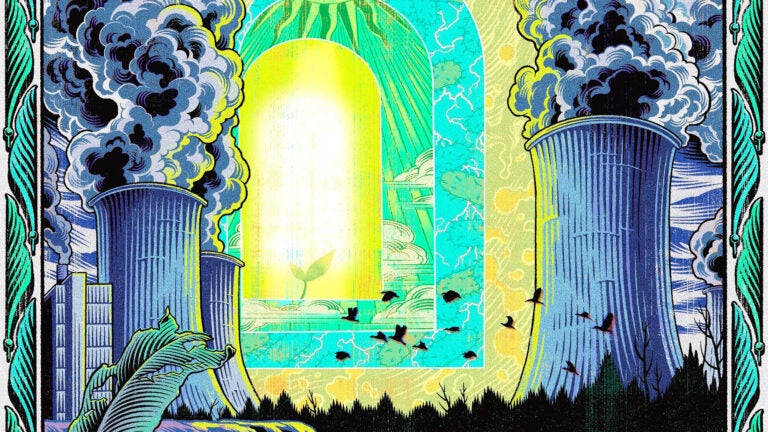
Oil Is for Fossils: The Future of Energy Lies in Nature’s Other Power Sources
Formed from the ancient remains of plants that lived and died millions of years ago, fossil fuels once seemed like supremely promising, benign gifts bestowed upon us by nature for our progress and prosperity.
Scientific ingenuity developed raw oil and coal into fuels for powerful engines that helped spur the greatest improvements in human living standards. More than that, they supercharged economies, transformed cities into bristling forests of skyscrapers, extended lifespans and landed men on the moon. They also came with a heavy cost.
These same fuels have polluted the atmosphere, contaminated ecosystems and poisoned our lungs. The emissions we create by burning them are on track to warm our planet to a perilous degree — unless we quickly change course.
Fortunately, nature offers potentially limitless alternatives to fossil fuels. Our sun beams down more energy in an hour than humanity uses in an entire year. And the soil beneath our feet is rich with tiny bacteria, silently conducting an electrical dance that dates back more than 3 billion years.
As scientists turn once again to the natural world for solutions to our energy needs, they are also taking precautions to avoid recreating a relationship as fraught as our centuries-old dependence on fossil fuels.
“We want to create a new paradigm, but not one that’s just as bad as the old one,” says Stephen Bradforth, senior advisor to the dean for research strategy and development and professor of chemistry. He is one of many researchers at USC Dornsife College of Letters, Arts and Sciences who are looking to nature to inspire scientific solutions and to provide green, reliable energy to meet our global needs.
Bacteria’s electric slide
In Moh El-Naggar’s lab, looking to nature requires a powerful microscope.
El-Naggar, divisional dean of physical sciences and mathematics, is exploring the curious qualities of a power-generating bacteria. As part of their metabolism, Shewanella oneidensis move electrons from the inside of their cells to surfaces outside their cells.
Building on the research of Professor Emeritus of Earth Sciences Kenneth Nealson, who first discovered these “electric bacteria” in the 1980s, El-Naggar says it’s possible to “wire-up” these bacteria to metal or semiconductor technologies to extract renewable energy, make biofuels or build new bioelectronics.
For instance, the bacteria could potentially be deployed in wastewater treatment plants where they would dine on what’s been flushed down the drain while providing the energy necessary to power the plant.
“In the United States, more than 5% of the energy from our electrical grid is used just to treat our waste,” says El-Naggar, Dean’s Professor of Physics and Astronomy and professor of physics and chemistry. “Technology that can treat waste while also generating its own electricity would obviously be a very welcome development.”
El-Naggar is hopeful his research will one day lead to “living electronics”: small devices, such as phones or lamps, powered exclusively by bacteria.
How do you hold a sunbeam in your hand?
Of course, bacteria are not nature’s only energy offering. The sun pumps out an astonishing 173,000 terawatts of power continuously — more than 10,000 times the world’s energy needs.
Solar panels enable us to capture some of this energy bonanza, but the silicon used to fabricate them is not a very efficient material, says Richard Brutchey, professor of chemistry. Its popularity, he notes, was driven largely by advances in low-cost production by China, which now dominates the solar panel market.
Game-changing new solar cell materials absorb light much more effectively than silicon, but these innovations use rare elements like indium and tellurium.
After digging through the rock catalog at the Natural History Museum of Los Angeles, near USC’s University Park campus, in search of a better alternative to conduct solar energy, Brutchey discovered a potential candidate: bournonite. Named after the French mineralogist Jacques-Louis, Comte de Bournon, this stable, black mineral is made of abundant elements, absorbs light well and can be printed into a thin film for solar panels.
We want to create a new paradigm, but not one that’s just as bad as the old one.”
There’s just one drawback
“It’s really promising, but it contains lead,” says Brutchey. “We’re investigating the possibility of substituting a non-toxic element for bournonite’s lead content.”
Brutchey isn’t the only USC Dornsife faculty member working on better, thinner solar energy technology. Visit Barry Thompson, professor of chemistry, in his office and he will unfurl a roll of thin plastic coated with a dark pattern of thin stripes. Each stripe is composed of organic molecules that can generate electricity from sunlight.
Because solar cells made of silicon require a lot of the material, they are bulky and rigid. The molecules
in Thompson’s organic solar cells could be inserted into materials flexible enough to bend, fold and even stretch. Plus, they are cheap to produce. With this sort of technology, Thompson thinks we could someday “print” solar panels. Long sheets of the molecules could be plastered over the exteriors of skyscrapers to generate energy for air conditioning, computers and water coolers.
And while we’re at it, why not do the windows as well?
Mark Thompson, Ray R. Irani, Chairman of Occidental Petroleum Corporation, Chair in Chemistry, is currently working on an exceptionally thin solar film that could cover windows and capture some 8% to 10% of the energy provided by sunlight falling on the building.
Thankfully, there is no need to worry that his idea would transform offices into inhospitable caves. The solar film does not affect the transparency of the glass. It also won’t dramatically boost costs, says Mark Thompson, professor of chemistry and chemical engineering and materials science. Coating glass with solar film would only slightly raise the cost of window production, and the increase would be offset by savings on energy bills.
This innovation could be a boon for cities like Los Angeles, which is aiming for all newly constructed buildings to be carbon-neutral by 2030. Rooftop solar panels won’t be enough to achieve that.
“When you get to buildings over two stories, there isn’t enough roof space for the panels necessary to generate the building’s energy needs. You have to add solar panels somewhere else,” says Mark Thompson. Adding his thin solar film to windows, and perhaps Barry Thompson’s printed solar sheets to building exteriors, could be just the trick to meet the city’s ambitious goals.
Sunshine on a cloudy day
Though the sun is a powerful generator, its energy can be partially — or even totally — inaccessible, depending on location, season and time of day. To entirely replace fossil fuels with sunshine, we need to solve our storage problem.
Lithium-ion batteries provide decent solar storage at small levels, but scaling up has been a major challenge. Once these batteries become large enough to contain the amount of solar energy necessary to power our industrial world, they will require complex, costly systems to avoid bursting into flames.
Plus, lithium-ion batteries use pricey metals such as nickel that (you guessed it) come from destructive mining in only a few locations on the planet. Our growing dependency on them could trigger similar geopolitical conflicts to those sparked by oil.
Scientists at USC Dornsife are devising better options. G. K. Surya Prakash, George A. and Judith A. Olah Nobel Laureate Chair in Hydrocarbon Chemistry, worked with the late Sri Narayan, professor of chemistry and co-director of the USC Loker Hydrocarbon Research Institute based at USC Dornsife, to develop redox flow batteries, which use chemical baths to store energy.
Charged electrons captured from solar panels are stored in one tank of chemicals. Once they have been used — to power a lamp for example — the electrons are returned to a different vat where they are recharged using solar power or other renewables, such as wind. It’s possible to scale up this process simply by adding more chemical tanks. Just as important, the systems have a low risk of catching fire.
Narayan and Prakash, professor of chemistry and chemical engineering and materials science, were figuring out how to make this technology rely on low-cost, readily available materials. In 2020, they demonstrated the success of a redox flow battery that uses iron sulfate and anthraquinone disulfonic acid for the chemical baths. Both materials are inexpensive and abundant.
Iron man
Redox flow batteries are a good solution to storing solar energy to use as electricity. But what about nonelectric energy needs? According to the Environmental Protection Agency, transportation accounts for almost 30% of greenhouse gas emissions in the U.S. Electric cars are gaining in popularity, but the batteries are heavy and require rare minerals. Plus, replacing every car and truck with an electric vehicle would generate an enormous amount of carbon during production and assembly.
Scientists think there’s a better way: hydrogen fuel. Hydrogen can store considerably more energy in less space than batteries, and refueling is quick. It’s also powerful. NASA already uses hydrogen to launch its rockets into space. Plus, existing combustion engines can be easily converted to use hydrogen instead of gas.
Currently, the cheapest way to make hydrogen is by burning coal. A greener way to manufacture it is through electrolysis, a process that sends electricity through water and splits hydrogen away from the oxygen molecule. The machines used for this, called electrolyzers, need expensive and rare materials such as nickel. This bumps up the price of production, making green hydrogen too costly to compete with gasoline — so far.
Narayan was developing a much less expensive way to build these machines to lower the price of hydrogen fuel by replacing all the nickel in the electrolyzer with inexpensive, iron-based materials. His all-iron prototype has already successfully racked up more than 1,500 hours of durability testing.
Liquid sunshine
There is another way to make green hydrogen: by copying nature. In photosynthesis, the sun’s rays spark a chemical reaction in plants that turns water and carbon dioxide into the sugars needed for growth.
Researchers want to mimic this process to generate hydrogen and other fuels. They aim to use the sun and precise chemical reactions to form hydrogen from water — with no electricity required. This approach, called photocatalysis, will need a little human assistance to meet our power needs.
Photocatalysis requires the use of a photosensitizer — a molecule that will absorb the sunlight — and the energy generated is used to kick-start the chemical reaction. Materials currently in use as artificial photosensitizers don’t take in much of the solar spectrum, which means they don’t convert as much of the energy available to us in sunrays. They also rely on rare, expensive materials like ruthenium and iridium.
Thabassum Ahammad, a doctoral student in chemistry under the supervision of Bradforth, is experimenting to see if Earth-abundant options for photosensitizers convert light as efficiently. He tests molecules that are designed and synthesized in Mark Thompson’s laboratory.
A visit to the lab reveals large tables on which a maze of spectrometers for measuring light is carefully arranged. When Ahammad flips a switch, a bright laser beam shoots out, bouncing swiftly across the surface of these instruments before hitting the sample of the test photosensitizers. Starting with the brief event of light absorption (faster than a nanosecond), the experiment captures, moment by moment, how well the photosensitizer is able to transform the energy from the captured light.
In addition to a photosensitizer, artificial photosynthesis requires special catalysts to help boost the production of energy. “Photosynthesis is actually not that efficient,” says Smaranda Marinescu, associate professor of chemistry.
“We’re interested in making this process faster and more productive.”
A chemical catalyst can be used to help speed up the process of generating hydrogen from water. Platinum is an excellent catalyst but — as anyone who has bought a wedding ring may know — it’s an expensive and rare metal.
Marinescu is looking for more abundant materials to create catalysts that are also more powerful than those available in the natural world. She’s currently investigating cobalt and sulfur-containing materials — both elements are much more commonly found than platinum — as promising and affordable options.
If all this innovation can produce cheap, cleanly produced hydrogen, fossil fuels could be replaced with relative ease. Plus, converting solar to fuel can help emerging nations and rural communities that may not have access to electricity. “If you can only convert solar to electricity, that doesn’t help people who are off the grid,” Ahammad notes.
The methanol cure-all
Using existing infrastructure to create a greener future is consistent with Prakash’s decades-long “methanol economy” work to replace fossil fuels with methanol.
An inexpensive wood alcohol, methanol can be mixed with water then dropped into a fuel cell to produce electricity.
It’s a clean-burning internal combustion fuel and avoids some of the complications of hydrogen, which must be stored as a pressurized gas and can diffuse into the atmosphere over time. Methanol, which can also be converted to all petrochemical products, could also use existing pipe infrastructure originally built for petroleum or natural gas transportation.
Most of the methanol in use today is created by using coal or natural gas, but “green” methanol can be made by capturing carbon from the air. In direct air-capture systems, air is drawn in by fans then cleaned of dust using filters. Next, it’s passed through a chemical solution that attracts the carbon dioxide, separating it from other molecules. Add some “green” hydrogen and catalysts to this collected carbon and presto — “green” methanol.
It’s a welcome way to clean the atmosphere of excess carbon dioxide caused by burning fossil fuels, while producing a green and renewable energy source.
“CO2 is not a bad molecule, we just need to manage it,” says Prakash. “Nature manages it through photosynthesis: we can manage it through chemistry.”
CO2 is not a bad molecule, we just need to manage it. Nature manages it through photosynthesis: we can manage it through chemistry.”
Plant power
Methanol and hydrogen represent just some of the cleaner-burning fuels out there. Off the coast of Santa Catalina Island, in the waters near the USC Wrigley Marine Science Center, lies a thick forest of kelp that USC Dornsife scientists hope could one day power airplanes and cars.
Unlike terrestrial crops, such as corn, which are grown for biofuels, kelp doesn’t need massive amounts of land, fresh water or fertilizer.
It is one of nature’s fastest growing plants, and the fuel derived from it doesn’t give off atmosphere-warming carbon dioxide. A Utah-sized patch of ocean kelp could potentially supply 10% of America’s energy needs.
Diane Kim, adjunct assistant professor of environmental studies, has been working on an ingenious mechanical contraption, dubbed the “kelp elevator,” which raises and lowers stocks of kelp to give them optimal access to sunlight and nutrients. A recent study found that this technique quadrupled production.
The future is (almost) now
Kelp fuel, skyscrapers clad with solar panels, electronics powered by tiny microorganisms — this might all seem a little farfetched. Don’t be too skeptical: USC Dornsife faculty already have an impressive track record of success.
A renewable methanol plant in Iceland, named for the late USC Dornsife chemist and Nobel Prize winner George Olah, an early collaborator with Prakash, already uses their innovation. It captures as much carbon as the local power plant emits and produces almost 530,000 gallons of methanol annually. And you might already be using technology invented by Mark Thompson to read this article. He came up with the molecules that display red and green colors on phone screens.
Could we soon power our world on sunshine falling through Mark Thompson’s solar windows, or fill our gas tanks with clean fuel brought to life by Marinescu’s catalysts? It wouldn’t be the first time scientists have been inspired by the wonders of nature to create revolutionary new wellsprings of energy.