Appetite for Change
Walking into the supermarket, browsing the seemingly endless rows of fresh produce and canned goods, it’s hard to fathom a food shortage. But with the current world population of 7.2 billion expected to explode to 9.6 billion by 2050, the United Nations warns food scarcity is in the wind.
Although urbanization will have gobbled up most arable land, more food will be needed. To cope with rising food insecurity, China is purchasing agricultural land throughout the world, in countries that include Russia, Mexico, Cuba and Australia.
The greatest spike will occur in developing countries, with more than half of the growth in Africa. By 2050, the U.N. reported, Nigeria’s population will exceed that of the United States, which is expected to balloon by 31 percent from 305 million in 2008 to 400 million in 2050.
“There’s a perfect storm on the horizon,” USC Dornsife Dean Steve Kay said. “We also have climate change that will stress our water resources. As we move from 2030 to 2050, it will be a challenging time for food security.”
It’s happening now in Central America, where drought conditions are wreaking havoc on crops. By early 2015, food security in four Central American countries is expected to rapidly deteriorate, according to an August alert sent out by the United States Agency for International Development’s (USAID) Famine Early Warning Systems Network.
To meet the food demand, agriculture production must at least double by 2050, the U.N. asserts, and “innovative strategies are needed to help combat hunger, which already affects more than 1 billion people in the world.”
Answering the call, researchers at USC Dornsife are finding innovative ways to feed the world. Some have built the foundation that may lead to the design of more robust crops. Others are tapping into aquaculture to bolster food from the ocean. Still others are homing in on palm oil and the small but mighty chickpea to help avert the world’s looming food crisis.
The Good Seed
The key word in the food security challenge is yield.
“How can we keep crop yields rising if traditional plant breeding isn’t working anymore?” Kay said. “We can now use genome sequencing or molecular markers to build more efficient plant breeding programs. Technology gives us a boost, but there’s absolutely no doubt in the minds of almost every expert that we will need agricultural biotechnology as well — or genetically modified foods.”
Kay, professor of biological sciences, and his research team have made giant strides toward constructing hardier crop plants — onions, beets, soybeans and the like.
In one major step, Kay and his team have created the first comprehensive library of genetic switches in plants, available for scientists to use around the globe. The collection contains about 2,000 clones of plant transcription factors, nature’s genetic on-off switches. Conducted over eight years, the research making the library possible was published in July in Cell Reports.
“They’re like smart missiles that go into the nucleus and bind to specific sequences of DNA,” Kay, the study’s corresponding author, said of transcription factors. “They will regulate genes, switching them on or off, according to how that cell needs to respond to its environment.”
Genetic switches are like turning on a light bulb so you can see in the dark. A switch will go on to allow a plant to withstand cold, for example, then go off when it’s hot.
In Kay’s library, clones of these “master switches” are contained in the “wells” of microtiter plates. The library will be sent to stock centers — or collections of living organisms — which will distribute the plates to scientists.
“We wanted to make a high-fidelity, gold-standard collection of transcription factors that’s going to serve the plant community all over the world,” said Kay, who researches circadian rhythms, or the biological clock, in plants.
The clones were taken from Arabidopsis, a flowering plant related to cabbage and mustard. The plant is used as a genetic model because it shares most of the same genes as many plants and crops.
“You can think of Arabidopsis as the mouse of the botanical world,” Kay said.
The availability of these clones has great implications for scientists such as Kay, whose research sets the stage to design a more robust plant for future food security.
“Ultimately, this collection will help us understand at the molecular level the mechanisms of how plants work,” said Jose Pruneda-Paz, co-first author on the paper. Pruneda-Paz helped to create the library as a postdoctoral researcher, first in Kay’s laboratory at The Scripps Research Institute in La Jolla, California, then at the University of California, San Diego (UCSD), where he is now a faculty member.
“By manipulating those transcription factors, we will be able to ultimately improve plant traits such as stress resistance or seed quantity and quality,” Pruneda-Paz said. “This is the larger goal.”
Kay elaborated: “Along the way we are going to understand the wiring — the instruction manual — for how plants grow and develop. From that knowledge base comes all the translational opportunities.”
The collection will help in the underfunded field of plant research. Of all biomedical research, the federal government spends approximately 1 percent on plant research.
“Given how important food is to human health, that’s rather concerning,” Kay said, adding that the goal is to build the foundation for any number of plant biology projects.
One study made possible by the library was published in Current Biology in July. In the research, Kay’s team investigated how plants regulate their gene expression in the cold. Using the library, they conducted tests isolating an interaction between two key genes — LUX and CBF1 — now known to be responsible for freezing tolerance in plants.
The research showed how plants adapt to temperature changes during the normal course of the day-night cycle, and to extreme change such as frost.
“We knew very little about how cold intersects with the clock and this really reinforced the idea that transcriptional regulation is key,” said Brenda Chow, referring to when a gene is triggered to respond to an environment. The first author on the Current Biology study, Chow recently started a position at GenBank, a genetic sequence database in Bethesda, Maryland.
“The library has been very useful across the plant community. For my project, it was a unique way to identify the interaction between CBF1 and LUX. It would have been very difficult to identify this any other way.”
Research on grasses, too, is paramount. Most human calories come from grasses — wheat, maize and corn. Kay’s team was the first to thoroughly characterize the impact of the circadian clock on grass growth.
In a study published in PLOS ONE in June, researchers from Kay’s laboratory revealed that internal cues generated by the circadian clock do not cause grasses to grow. Regardless of the presence or absence of light, grasses grow in warm temperatures.
“It’s known that things grow faster when they’re warm and enzymes work faster when they’re warmer; temperature is a catalyst,” said the study’s first author, Samuel Hazen, now a faculty member at the University of Massachusetts, Amherst. “But the complete absence of the involvement of light/dark cues in grass growth is what we discovered in the study.”
This breakthrough is highly relevant to researchers who, as Kay said, can use the knowledge as the base for translational research. For example, it becomes the foundation for scientists to modify genes in grasses to withstand drought and other adverse weather conditions.
In two more recent studies, published in the Proceedings of the National Academy of Sciences (PNAS), Kay and his collaborators identified genes and pathways crucial for plant growth under stressful conditions, such as heat and lack of nutrients.
In one study, Kay’s team discovered a key molecular cog in a plant’s biological clock — one that modulates the speed of circadian (daily) rhythms based on temperature. Postdoctoral scholar Dawn Nagel identified transcription factor FBH1, revealing a novel role for this switch in the plant’s ability to respond and grow in warm temperatures. This discovery provides clues to managing crops under high-temperature stress.
And in collaboration with professor Nigel Crawford of UCSD, Kay’s lab identified another genetic switch, TCP20, important for how roots forage and sense nutrient levels. Understanding root growth under limited nutrient availability, particularly nitrogen, is vital for developing crops that lack this important nutrient source.
Kay also noted that while some claim genetically modified organisms (GMOs) may be unsafe for human consumption, he believes this stance is based on suspicion rather than evidence.
“It comes from a lack of understanding,” he said. “With more transparency and facts, the public will come to see GMOs as a solution.”
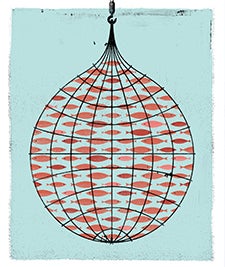
The United States imports 91 percent of its seafood, half of which comes from farms. While many countries are investing heavily in sustainable aquaculture, the U.S. ranks last in aquaculture production. USC Dornsife scientists are finding ways the U.S. can provide more seafood.
Angling for Answers
Moving from land to sea, aquaculture is also a way to help feed the world.
As oceans are reaching their maximum potential of wild, captured seafood, fish farming has become the world’s main way of producing species such as trout and salmon, according to the U.N.’s Food and Agriculture Organization.
Over the past 20 years, the world’s wild-fish catch has flattened, with the depletion and threatened extinction of some species. Meanwhile, fish farming continues to rise at a sharp rate.
Fish farming — the breeding, rearing and harvesting of plants and animals in ponds, rivers, lakes and the ocean — is now providing more than half of the world’s 44 pounds per capita of fish consumed annually. (In the U.S., annual consumption is 15 pounds per capita, compared to 110 pounds per capita of red meat).
In 2012, the amount of farmed fish produced globally was at an all-time high of 90.4 million tons. But the U.S. ranks last in aquaculture production. China ranks first, producing 59.7 percent of all farmed seafood. Indonesia comes in second with 10.6 percent, followed by India with 4.7 percent. The U.S. is responsible for 0.5 percent of farmed production, just below North Korea.
Currently, the U.S. imports 91 percent of its seafood. Looking to our own coastlines to provide seafood is economically and socially imperative, said Dennis Hedgecock, Paxson H. Offield Professor in Fisheries Ecology and professor of biological sciences at USC Dornsife. Along with Donal Manahan, professor of biological sciences and vice dean for students, Hedgecock is working on establishing a one-of-a-kind facility for complete life-cycle culture of model marine organisms at the USC Philip K. Wrigley Marine Science Center on Catalina Island.
The culture facility would become a national stock center for pedigreed lines of Pacific oysters and purple sea urchins — marine invertebrates with sequenced genomes. They may culture other species such as Kumamoto oysters, Mediterranean and California mussels, and purple-hinge rock scallops.
Hedgecock acknowledged that fish farming in the U.S. has been sorely misunderstood, with environmentalists concerned about pollution caused by on- and off-shore farms, and consumers worried about taste and nutrition. Permits can be tough to obtain.
“Aquaculture is the future,” Hedgecock said. “If there are problems, we have to solve the problems. We already get our seafood from other countries where we have no say in what environmental damage they may cause. It’s irresponsible to push off our consumption to other countries.
“Why don’t we learn this process ourselves and be leaders instead of followers?”
Along the U.S. West Coast, some shellfish farms are economically and environmentally thriving. To help promote aquaculture research, Hedgecock, Manahan and biologist Dale Kiefer have spearheaded a new initiative at the USC Wrigley Institute for Environmental Studies called “Future of Food From the Sea.”
This research is unlocking many of the genes involved in oyster growth and survival, helping scientists and aquaculturists understand and improve the relationship between oyster genetics and production. Hedgecock and Manahan’s research relies on hybrid vigor — or heterosis — to produce oysters resistant to environmental stressors. In research published in PNAS and other publications, the scientists have shown how crossbreeding genetically different oysters to create hybrids increases size, yield and other positive characteristics.
Right now, Hedgecock and Manahan operate an oyster hatchery on Catalina, but aim to create a facility that can accommodate the oysters through adulthood, enabling researchers to further understand how these important species survive, grow and adapt.
Hedgecock said that while the genomes of a growing number of marine organisms have been sequenced, few have the well-developed genetic resources and methods of model terrestrial animals.
Most research on marine species relies on wild-type animals taken directly from the field, obtained from biological supply houses or the aquaculture industry. This practice contributes to variation in results among laboratories and, more importantly, prevents a sophisticated partitioning of genetic and environmental causes of variation in complex phenotypes, he said.
“Earth’s largest habitat, the ocean, is undergoing rapid anthropogenic change,” Hedgecock said. “The ocean is warming, becoming more acidic as a result of anthropogenic carbon dioxide; toxic, plastic particles and other wastes; and heavy exploitation for mineral resources and food for human consumption.”
Understanding and predicting biological stability and change amid rapid anthropogenic modifications of ecosystems is a grand challenge facing environmental life scientists, he said.
“In the short term, organisms withstand environmental stress, including disease,” he said. “In the long term, across generations, populations evolve using primarily genetic variation in traits that increase fitness. Separating nature and nurture is key to understanding the potential for adaptation to future environmental change.”
Helping to make the hybrid vigor research possible are donors including Sam King, a member of the USC Wrigley Institute Advisory Board, the USC Dornsife Board of Councilors, and chief executive officer of King’s Seafood Company. King, Hedgecock and others give talks throughout the nation on the institute’s aquaculture research.
“I think our attitudes are starting to change,” King said. “As long as we have great scientists and great science, then hopefully the minds of American people will change. Like people in other countries, they’ll learn to embrace aquaculture.”
Sergey Nuzhdin, professor of biological sciences at USC Dornsife, is working with Hedgecock on some of the aquaculture research. Nuzhdin’s laboratory has long been interested in tackling food insecurity.
In 2008, Nuzhdin received a $3.2-million National Science Foundation grant to fund a three-year study on the legume Medicago truncatula, a plant that can improve soil fertility naturally, even under saline conditions.
The species is a genetic model for crop legumes such as soybean, alfalfa, pea, bean and peanut. The principal investigator for the cross-cultural study, Nuzhdin collaborated with researchers from Tunisia and France.
From that study, other projects have evolved. Nuzhdin became a key member of the Chickpea Innovation Lab, among 23 “innovation labs” participating in USAID’s global hunger and food security initiative, Feed the Future.
The project is led by professor Doug Cook of the University of California, Davis, with major collaborators at USC, Florida International University, Turkey’s Harran University and the Ethiopian Institute of Agricultural Research.
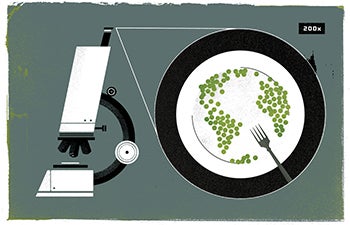
Generating higher crop yields, genetically modified foods may be an answer to easing world hunger.
Eat Your Peas
USAID’s five-year, $4 million genetic research program — with $1 million slated for USC Dornsife — aims to expand the climatic range in which the chickpea can be cultivated. By creating more and higher yielding varieties of chickpea, the productivity of small-scale farmers will also increase.
In early 2013, Nuzhdin and his colleagues conducted research in Turkey, where some 10,000 years ago people turned wild plants into crops — the advent of agriculture.
Also called the garbanzo bean, the chickpea is extremely high in protein and its natural ability to fix atmospheric nitrogen contributes to soil fertility. In developing countries, the crop provides a crucial source of income, food security and nutrition to poor farmers.
Nuzhdin and his fellow researchers are capitalizing on the genetic potential of the wild chickpea to create traits that withstand heat, drought, frost and disease, and encourage increased nutrition.
Ethiopia, the largest chickpea producer in Africa, was chosen as the thematic focus of the project because of its history of chickpea cultivation stretching back more than 2,500 years. As a result of their religious practice of fasting, many Ethiopians have a high dependency on the chickpea as a source of protein.
Ultimately, the chickpea plants will be tested in the U.S., Canada, Australia, Turkey and Ethiopia. Nuzhdin’s research has the potential to improve chickpea breeding for the next century.
In another project, Tatiana Tatarinova, associate professor of research pediatrics at Children’s Hospital, Los Angeles, and Nuzhdin have teamed up on research supported by the Malaysian Palm Oil Board (MPOB) — a Malaysian government research organization. The team will help develop tools to accelerate the interspecific hybrid breeding of oil palm trees.
An MPOB collaborator since 2010, Tatarinova was drawn to the group’s vast collection of high-quality, diverse, biological information on oil palm.
“For a bioinformatician, it’s like a trip to Disneyland,” Tatarinova said. “It’s a rare chance to get access to an unexplored wealth of data that can have immediate practical applications for agriculture.”
Oil palms produce an edible vegetable oil derived from the reddish pulp of their fruit. Palm oil has become among the world’s major sources of vegetable oil, a common cooking ingredient and butter substitute.
Nuzhdin and his team will study Elaeis oleifera, a species of palm native to South and Central America, and compare it to Elaeis guineensis, an African oil palm. In view of E. oleifera’s lack of traits linked to high oil yield and E. guineensis’ high oil yield, researchers will help to develop tools to quicken interspecific hybridization of both species.
“We’re interested in using modern genomics tools to improve the oil palm tree,” Nuzhdin said. “Artificial selection plays a central role in making those improvements. But selecting alone will be too slow for the oil palms, since they grow for 10-plus years before starting to reproduce.”
His team will use the power of biodiversity in this effort. Rather than genetically modifying an organism, they will use the tree’s genetic makeup to predict its agronomy and traits.
“We use principles of evolution and ecology to understand what is important and then enhance what is natural,” he said.
He acknowledged that while palm oil is the main component of all processed foods, cosmetics and cleaning products in the U.S., harvesting the trees to make the oil has raised concern regarding deforestation and species extinction. While the debate continues, Nuzhdin’s research will boost breeding efficiency and help make the production of palm oil more sustainable.
“Since many economies, like Malaysia’s, completely depend on oil palm production, they are not about to stop producing the trees,” Nuzhdin said.
“The only way of reducing the impact is to intensify the production on already existing fields, instead of extending to new land. Breeding the trees that can produce faster and more reliably will mitigate any damage to the environment.”
Applying the research to the real world is essential, he said.
“We have a set of deliverables,” Nuzhdin said. “Those deliverables are cultivars that will get into the hands of small farmers in developing countries.”
Senior writer Susan Bell contributed to this report.
Read more stories from USC Dornsife Magazine‘s Fall 2014-Winter 2015 issue