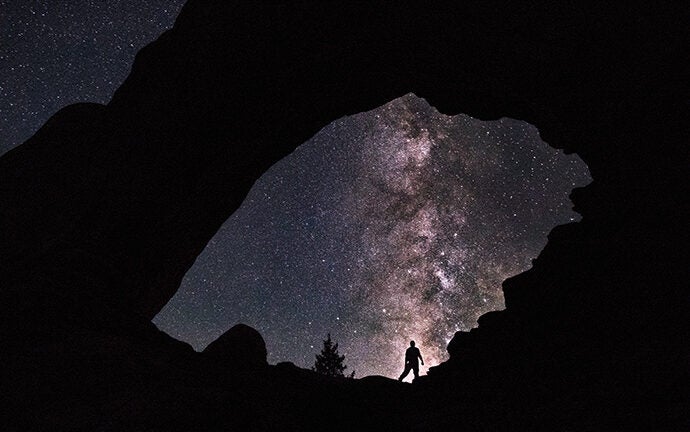
The Universe As We Know It
Sitting in a small French bistro across from Pershing Square in downtown Los Angeles, Clifford Johnson held the pumpkin-hued drinking straw parallel to the table.
“Essentially, this straw has two dimensions to it. I can walk along the straw,” he explained, running his index finger along the length, “or I can walk around the straw.” His finger traced the object’s glossy curved surface.
Johnson, professor of physics and astronomy at USC Dornsife, was explaining how the universe could hold extra, hidden dimensions.
“But let’s imagine the straw is really, really long and thin, and I’m walking along the length and I don’t have the equipment to look at very, very small distances.” With those limitations, a traveler would only be able to see the one dimension of length ahead and behind.
Johnson motioned toward a point past the noisy traffic and pedestrians passing by, across the street. “It’s basically the same as if I took the straw over there. You would see its orange length, but you wouldn’t see the roundness. Still,” he said, his finger again circling the straw, “notice everywhere I am in the one-dimensional universe, I could go around if I wanted to. That extra dimension is always with me.”
In a similar way, the four-dimensional universe that humans know, comprising three dimensions of space and one of time, could actually harbor other dimensions that are too small to detect, Johnson said.
Why is that important? One word: strings.
Johnson, who describes his research as an attempt to understand the basic fabric of nature, is a renowned expert in string theory, the closest thing scientists have to a single theory that explains everything in the universe — all of reality.
If he and his fellow physicists are right, strings may be the most basic unit of existence. Every particle of force or matter may boil down to a simple, one-dimensional, vibrating string.
The large and small of it
For most of history, humankind’s view of the universe and how it works focused on large-scale phenomena — planetary motion, visible properties of light and effects of magnetic fields, for example. Around the turn of the 20th century, as physicists began to examine the microscopic universe of atoms and their constituent pieces, they found that the subatomic world seemed to be governed by a very different set of rules. Max Planck, Albert Einstein, Niels Bohr and a host of brilliantly creative scientists began exploring this realm through mathematics and direct experimentation.
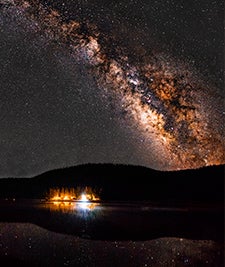
The universe, while incomprehensibly large, may boil down to infinitesimal basic building blocks.
As the scientists worked over the next several decades, they discovered that there were two different classes of fundamental particles, fermions and bosons. The former are the core constituents of matter while the latter mediate interactions between pieces of matter.
Stated simply, different kinds of bosons transfer forces between different kinds of fermions. Photons, for example, transmit the electromagnetic force between charged fermions such as electrons.
“This major breakthrough — that there are particles that can communicate forces or interactions — that was an amazing piece of quantum physics that was understood in the middle of the last century,” Johnson said.
This quantum system seems to work nicely for three of the four known forces of nature — the strong nuclear force, which holds the particles in the atomic nuclei together; the weak nuclear force, which strains to break those nuclei apart as radioactive decay; and electromagnetism.
In other words, these subatomic forces fit a single, unified theory of quantum physics.
The one force that seems to resist following quantum rules — and therefore confounds a unified theory of everything — is gravity.
A curvaceous conundrum
Einstein famously described gravity as a curvature in the fabric of space-time. His revolutionary general theory of relativity — which marks its centennial anniversary in November 2015 — seems to work almost entirely on large scales (the level of planets, stars and galaxies) and at low energies. It breaks down in the tiny, high-energy spaces where bosons and fermions play.
Put another way, quantum physics works best where gravity is ignored, and general relativity works best on large systems — far above the subatomic scale — where quantum effects are very small.
In addition, physicists have yet to find a particle that conveys the force of gravity — the so-called graviton.
“We think it’s inevitable that there’s something like a graviton if you quantize gravity, and we’d be surprised if gravity isn’t quantum mechanical,” Johnson said. “The fact that we haven’t succeeded in doing it yet is our failure, not nature’s.”
The attempt hasn’t been completely fruitless, however.
Pulling strings
In the late 1960s and early ’70s, physicists began to take a different look at the bosons and fermions in the nuclei of atoms. They found the particles involved could be described as incredibly small, one-dimensional, vibrating strings.
This string theory quickly captured attention, but just as quickly fell out of favor as other models of particle interaction arose. This oscillation in interest would continue for some time.
“[The theory] has gone in and out of favor for quite a few years,” explained Nicholas Warner, professor of physics and astronomy and mathematics. “It was first invented as a theory of the strong interaction, and it was a failure at that. But then it resurged in the ’80s as a theory of quantum gravity, and it works extremely well.”
In fact, one very important observation stood out from early on — these vibrating strings were able to describe, to a T, the expected properties of gravitons.
“The coolest thing about string theory is it’s the only theory out there that reconciles quantum mechanics and general relativity,” said Warner, who uses string theory to understand the quantum physics of black holes, the most gravitationally intense phenomena in the universe. “It does seem to do that to the extent we’ve been able to calculate.”
Those calculations require a key caveat, however. The universe must contain extra dimensions.
Beyond 4D
Fortunately, extra dimensions need not be a problem. As Johnson illustrated with his orange straw, the universe may contain countless dimensions that are just too small to detect. But, because strings also are incredibly minute — smaller than anything previously conceived — and only one-dimensional, they can vibrate in essentially any of those dimensions. That’s important, because while string theory works very well to describe all of the observed particles — and even gravitons — it only succeeds if the strings can vibrate in as many as 10 dimensions.
“When you start writing down the mathematics, the strings come back and they tell you that the mathematics isn’t going to work out unless you allow us the freedom to vibrate in other dimensions as well,” Johnson said.
“When you allow the strings to be extra-dimensional … then it gives you a much richer range of possibilities, and the possibility that you can incorporate everything we’ve observed into string theory suddenly becomes viable,” he added.
In other words, each specific particle can be described as a string vibrating in a particular dimension.
“String theorists are trying to say there’s only one basic kind of particle and everything is just some different vibrational state of the string,” Warner explained. “The graviton is one fluctuation or vibration of the string, the photon is another … and so forth.”
So in the end, everything may boil down, essentially, to the same basic thing — strings.
Except, maybe not …
While string theory is remarkably adept at potentially describing all of the known particles of matter and force, it has yet to be tested.
“There’s always the possibility that the framework is incomplete, or just plain wrong,” Johnson said. “We need a way of getting measurable predictions from the theory that we can go away and test — a key step in any scientific endeavor.”
Strings, however, are likely too tiny to be seen directly by any experiment that scientists can hope to design soon, he said. So they need to look for indirect signs of strings, and string theory so far is not understood well enough to predict what those signs are.
But there is hope. String theory may get an indirect test when applied to what appears to be the most abundant material in the universe.
Observations show that dark matter and energy constitute more than 95 percent of the universe. Scientists have established that they are new forms of matter and energy, but so far their precise nature is unknown. They may hold the key to confirming the veracity of string theory, Johnson said.
“It’s really kind of amazing — and humbling. There are forms of matter that seem to show up naturally in string theory that could well be good candidates to be dark matter,” he said. “People are hoping that this could be a key to making contact between theory and nature.”
Learn more about the intricacies of mysterious black holes.
Photos by Jesse Thornton for USC Dornsife Magazine
Read more stories from USC Dornsife Magazine‘s Fall 2015-Winter 2016 issue >>