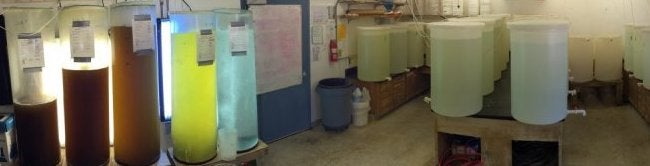
Larval culturing
Large-scale mariculture of larval bivalve and echinoderm. A culture vessel contains millions of larvae in 200-l seawater. I also have experience on recirculating culturing systems for fish and amphibian larvae.
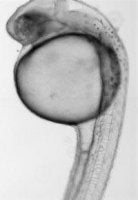
Whole-organism level measurements.
My work adapts a top-down approach, with observations first made at the whole-organism level. For example, an often measured trait is larval growth. Growth rate is assessed as changes in size, mass, and biochemical contents. Larval mass is further partitioned into total mass, ash mass, and organic tissue mass. Organic mass is classified into protein, lipid, and carbohydrate. From these measurements, larval energetic budget can be calculated.
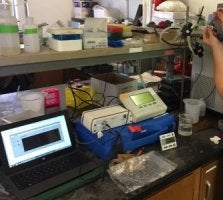
Physiological rates
My main research interest is animal physiology (“How Animals Work”). I have employed a wide range of physiological measurements to answer questions related to animal energetics. These measurements can be classified into energy acquisition processes (rates of feeding and nutrient uptake), oxygen delivery processes (rates of ventilation and cardiac activity), and energy consumption processes (metabolic rate that is further partitioned into its causal biochemical components).
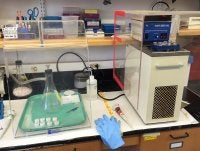
Biochemical rates
Many major energy-consuming processes lie at the biochemical level. My work has shown that protein synthesis, sodium-potassium transport, and calcium transport collectively consume the majority of the cellular ATP available to a larva. These measurements involve the use of radioactive tracers to label proteins and ion flux. Energy equivalents of these biochemical processes can then be calculated based on stoichiometry, or measured using specific inhibitors.
Cellular immunostaining
Cellular localization of mRNA and protein helps elucidate where the measured physiological and biochemical rate processes take place in the organism. Image: (A) fluorescent immunostaining of sodium-potassium pump protein in a 72-h-old larva of the zebrafish (head pointing up). The sodium-potassium pump is a marker for ion-transporting cells. (B) A close-up view of the ion-transporting cells with sodium-potassium pump protein localized in the plasma membrane. (C) The same field of view as in Panel B, but with double staining of sodium-potassium pump protein (green) and calcium channel mRNA (dark blue).
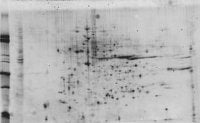
Molecular biology
Expression levels of genes and proteins go hand in hand with the in vivo measurements of rate processes and localization of mRNA and protein to explain environmental adaptation and phenotypic variation at different biological levels. An understanding of the molecular mechanism also helps develop potential molecular markers for the prediction of organismal, physiological, and biochemical traits. Image: two-dimensional gel analysis of proteome in fruit flies.